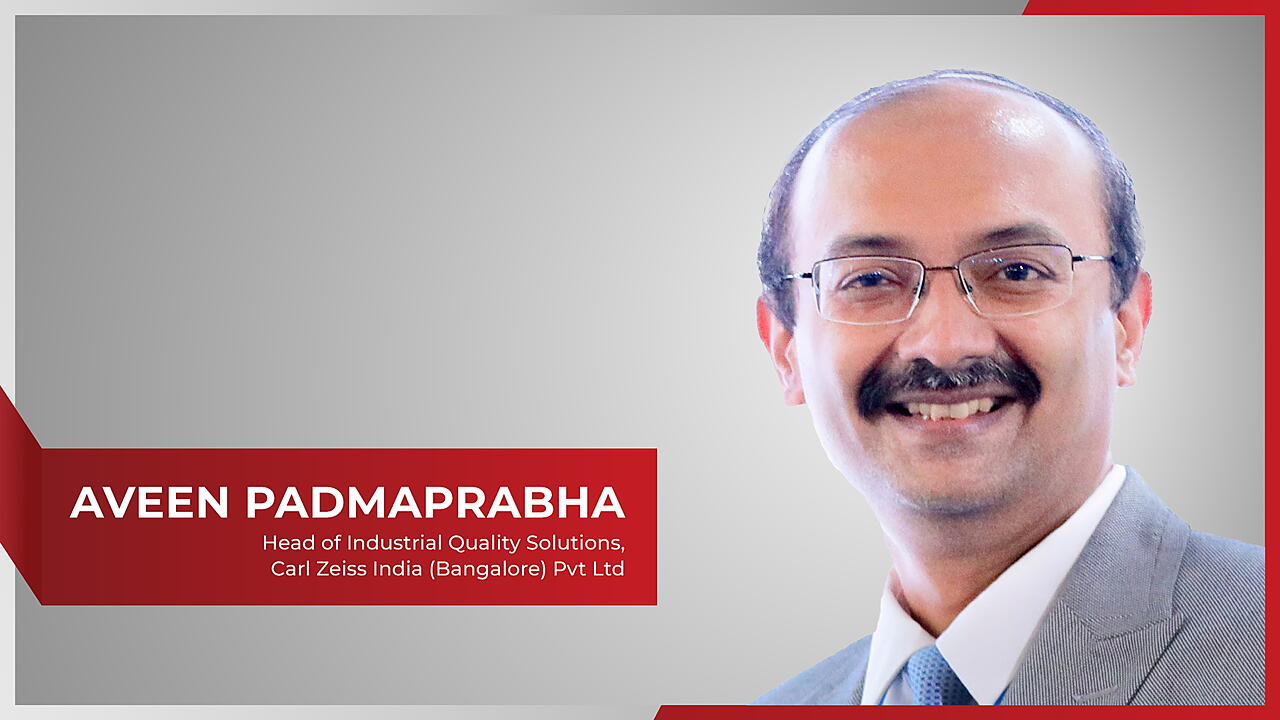
The rising BEV demands require a multimodal shift in manufacturing and QA practices for all manufacturers. We're still importing most components associated with the battery ecosystem, and there is a dire need to develop the capabilities or alternatives in India. In an insightful discussion with Aveen Padmaprabha, Head of Industrial Quality Solutions at Carl Zeiss India (Bangalore), we delve into the significant impacts of the surge in electric vehicle (EV) production on the automotive industry, particularly focusing on manufacturing and quality assurance. Padmaprabha provides a detailed overview of the challenges and innovations in EV battery technology, highlighting the critical role of quality assurance in this evolving landscape.
With a large number of EVs being made in India, what are the significant impacts of this surge on the automotive industry, particularly in terms of manufacturing and quality assurance?
Battery electric vehicles (BEVs) are the most prevalent type of non-hybrid EVs. They utilise batteries to store electrical energy that can be accessed at any time. Most EV batteries are lithium-based since they have a minimal discharge rate, and their energy density, both with regards to weight as well as to volume, is unparalleled by any other widely available electrical energy storage technology. However, 56% of natural lithium resources are located in South America – within Brazil, Argentina, and Chile. China controls more than 8% of the available lithium resources worldwide. Currently, India imports more than 70% of its lithium from China, as it has more than half of the world's lithium refining capacity. Whether this changes with the newly identified natural lithium reserve of an estimated 5.9 million tonne in the Salal-Haimana area in Jammu and Kashmir remains to be seen.
As a response to the resource dependency as well as an effort to address the need to electrify large transport vehicles, we witness a surge in the research of both alternative battery materials as well as alternative EV technology such as fuel cell EVs (FCEV) in India. The government has heavily increased its incentives for green hydrogen and electrolyser manufacturing in early 2023, and it is estimated that we will see the first hydrogen-fuelled trucks on Indian roads by 2030. However, the current focus is on BEVs.
There is a significant difference in the number of components between traditional internal combustion engine vehicles (ICEs) and EVs. BEV drivetrains have only six main components: the battery pack, a power inverter, an electric motor, an on-board battery charger, the Battery Management System (BMS) and a charging port. ICEs, in comparison, have thousands of parts and hundreds of moving components. The overall number of moving parts built into an EV is only 10% of that built into ICEs. This ultimately affects the manufacturing and quality control process.
Knowledge about physics and maintaining tight tolerances and precision is still crucial for EVs, especially when it comes to the e-motor with its fine hairpins in the stator. Technologies such as Coordinate Measurement Machines (CMMs), in combination with cutting-edge optical metrology, boost efficiency and ensure reliable quality. However, it also becomes increasingly important to understand material science and battery microstructure. Therefore, metrology solutions such as CTs and X-Ray gain relevance, as it is vital for failure analysis or quality control to be able to look inside the cell and even generate a comprehensive 3D-model for analysis and comparison. Lastly, microscopy can be utilised not only for R&D purposes but also to check the quality of raw materials.
How does understanding the microstructure and identifying potential sources of contamination play a crucial role in enhancing battery quality and, consequently, the overall performance of EVs?
Understanding the microstructure and cell chemistry of batteries is crucially important to enhance both performance, reliability and safety. The active material placed on the cathode is usually a combination of either nickel, manganese and cobalt (NMC) or nickel, cobalt and aluminium (NCA). These materials offer high performance when interacting with lithium and are relatively lightweight. However, cobalt is a conflict mineral with deposits concentrated in the Congo, leading to an unsustainable and highly dependent supply chain. An alternative material combination is lithium iron phosphate (LFP). This cathode material is heavier and less performing than NMC and NCA, but it is more readily available, not dependent on one single country alone, and cheaper to procure. The thorough research of any material's characteristics is vital to understanding the potential for efficiency and improvement in performance as well as maintaining safety and reliability.
Whichever cathode material is being used, there are possibilities of contamination that need to be avoided. Chemical contamination – other compounds apart from NMC, NCA, or LFP present in the cathode material – can lead to unwanted chemical side effects such as gas formation or heat development. Mechanical contamination – an uneven distribution and particle size of the material – not only leads to inefficiency but carries the risk of damaging the separator membrane, leading to shorts and even combustion of the battery.
Researching both the materials and microstructure of batteries and consequently monitoring the manufacturing process with regards to contamination, material quality and precision, is vital for advancing battery performance as well as battery quality.
Battery range, performance, and longevity influence consumer decisions in the EV market. What implications does this have for manufacturers in terms of design and production priorities?
In India, most consumers are concerned about charging time, range, cost and safety. These issues can be addressed by manufacturers of lithium-ion batteries by taking two primary factors into account when designing EV batteries: cathode materials and cell shape.
Manufacturers usually choose between NMC, NCA or LFP. LFP is cheaper and easier to procure while maintaining a sustainable supply chain. It also has a higher cycle life and functions more reliably even under hot temperatures. However, it has a lower energy density than NMC and NCA and is therefore heavier and occupies more space. So, if manufacturers emphasise performance over longevity, they mostly opt for NMC or NCA. NCA tends to have a slightly higher lifespan than NMC due to its lack of manganese. However, it is rarely used nowadays because it is less stable than NMC.
Regarding the shape of batteries, there are three main types to consider: cylindrical, pouch, and prismatic. Among these shapes, prismatic batteries have the highest cell capacity per volume, followed by pouch and cylindrical shapes. For this reason, EVs mainly use prismatic batteries. However, if flexibility and space optimisation in battery pack design are the priorities, pouch-shaped batteries are the most versatile. This is because they have soft aluminium coatings, making them lighter and increasing their energy density. Cylindrical designs are the most common ones used in various consumer devices, such as remotes. Their manufacturing process is highly optimised but due to their shape, their space utilisation is limited. They also reached their limit in energy density, still making them an asset for household and commonly used devices, but less suitable for EVs. We witness that most EV manufacturers nowadays focus on range and performance and, therefore, opt for prismatic shapes due to their high capacity. However, the battery research community is very active. Looking ahead, the increase of efficiency and capacity in pouch cells might eventually better meet the demands for EVs in terms of performance and range than prismatic cells in the future. In terms of longevity, pouch and prismatic cells can endure an equal amount of charging cycles.
With regards to addressing safety and cost concerns, LFP in pouch or prismatic shapes is the obvious choice for manufacturers. However, range anxiety and charging time conflict with that, as NMC is the best choice to reassure customers in this regard.
Can you elaborate on the critical role of high-quality materials and precision in production techniques for battery manufacturing? How do these factors directly impact the final product's performance and reliability?
A major cause of safety incidents in EVs is related to cell defects and degradation. Cell defects relate to leakages, short-circuits, dendrite growth, and more, whereas cell abuse means external extrusions, exterior physical damage to the cell, and over-charging or -discharging. In the worst case, these failures can lead to spontaneous combustion. Ensuring high-quality material and precision in production techniques help to prevent these defects. Therefore, a holistic quality assurance process from raw materials to exhaustive testing must be implemented to detect even the smallest defects, as they may eventually lead to battery failures.
The material quality control process is just the first step. In that stage the raw materials are analysed in terms of particle size and distribution as well as purity. The material must be evenly distributed across the electrode foil and not be contaminated to avoid any unwanted side effects that can impact the performance and longevity as well as the reliability and safety of the battery. This step further entails the production process control as well as the final product inspection and testing.
The next step of the quality control process is testing the cells in a controlled environment that ensures safety in a variety of situations. Typical testing scenarios include individually charging / discharging under well-known parameters as well as collision and battery short-circuit testing. This enables battery manufacturers to certify their cell quality to their customers, increasing trust.
Further, one must make sure that the BMS works sufficiently as it monitors the battery's status, its temperature and charging and discharging status.
Lastly, a fault diagnosis system detects problems and provides corresponding solutions promptly.
The manufacturing of the battery tray, which contains all the battery cells, connectors, and control units, necessitates a high degree of precision. The tray must be designed to endure the thermal expansion of the battery pack during charging and operation. It also needs to be seamlessly integrated into the car's structure due to the substantial weight and energy stored in the battery cells, as well as to guarantee the battery's structural integrity in case of an accident. Given that the battery tray is a safety-critical component often subject to 100% monitoring, an inline production solution is needed. This is in addition to the reference measurement in the measurement room, where the most critical elements are measured.